Brain stimulation against stress disorders?
One day it may be possible to cure brain disorders quite literally in our sleep – without taking pills
ETH researchers are using brain stimulation to investigate the consequences of stress and to find new therapies for treating stress-related psychiatric diseases. One day it may even be possible to cure brain disorders quite literally in our sleep – without taking pills.
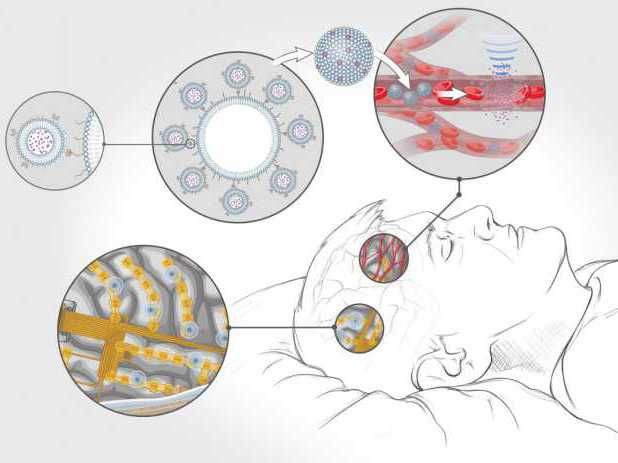
Vision of the future – Chips implanted in the cerebral cortex measure brain activity and activate drugs locally as and when required.
Jemère Ruby, based on a concept by Mehmet Fatih Yanik
Some videos on the internet are remarkably striking. In one, a man is sitting on the sofa holding a remote control. His hands and arms are trembling and shaking in a way that clearly indicates Parkinson’s disease. He lifts the remote control, points it at his chest and presses a grey button – and his tremors subside almost immediately. What we don’t see in the video are the two electrodes in the patient’s brain connected to a pacemaker implanted in his chest. When he presses the button, the pacemaker delivers electrical pulses to the basal ganglia, a group of neurons involved in movement planning and control. Stimulating this brain area, which is heavily affected by Parkinson’s disease, causes the motor symptoms to cease almost immediately – producing an almost spooky effect.
“Deep brain stimulation for Parkinson’s disease may well be one of neuroscience’s greatest success stories,” says Johannes Bohacek, an assistant professor at the Institute for Neuroscience at ETH Zurich. Researchers are also experimenting with brain stimulation as a means of treating depression, but much of the research is still in its infancy – and some still seems like pure science fiction.
Introducing viruses into the brain
Bohacek uses brain stimulation to investigate how stress affects the organism. “Acute and chronic stress are risk factors for mental disorders,” he says. Yet unravelling stress responses is a highly complex business, he notes, because stress affects not only the brain, but the entire body, involving many organs, hormones and neurotransmitters. “That makes it harder to conduct targeted research into stress as a specific phenomenon.”
That’s why Bohacek has opted to simplify things by focusing on individual elements. He is currently investigating the noradrenergic system, which plays a key role in stress. In situations of acute stress – for example when a fire alarm suddenly goes off – the brain is flooded with norepinephrine. The principal site responsible for synthesising norepinephrine is a tiny area of the brain called the locus coeruleus. This is hidden away deep in the brain stem rather like a needle in a haystack. “It is far too small and difficult to access for the kind of probes we use for deep brain stimulation,” says Bohacek. An overactive locus coeruleus can cause certain types of anxiety and panic disorders, which is why many scientists and the pharmaceutical industry are so determined to gain a better understanding of how it works.
In order to ascertain what happens in this particular brain area in stressful situations, the ETH professor uses designer viruses to specifically modify the activity of neurons in the locus coeruleus. To do this, he works with a special mouse line that restricts expression of the virus selectively to the locus coeruleus. The virus then causes an artificial receptor (receptor molecule) to form on the surface of the nerve cells, and specific substances can activate this receptor, much like a single key fitting a specific lock.
By activating these receptors, the researchers can trigger the release of norepinephrine without the need to activate the entire stress system. This allows Bohacek and his team to then study the changes that unfold in response to noradrenaline release in the entire brain.
The neuroscientist hopes these kinds of experiments will lead to a better understanding of the causes of stress disorders. “In order to develop more effective therapies, we first need to gain a greater insight into the molecular stress mechanisms. Regulating the excitability of locus coeruleus neurons using similar methods to brain stimulation would be a very interesting approach,” says Bohacek. “But it remains to be seen if and when these techniques will finally become a clinical reality.”
Brain machine interfaces
New therapies for brain disorders are also at the top of Mehmet Fatih Yanik’s agenda. “We’re working on technologies to fix network dysfunctions in brain disorders. Such dysfunctions are at the heart of many brain disorders ranging from depression and schizophrenia, to autism,” says Yanik, Professor of Neurotechnology at the Institute of Neuroinformatics at ETH and the University of Zurich.
Pills are still the most common way to treat brain disorders. The active substance in the medication binds to the corresponding target molecule in a nerve cell, typically triggering or inhibiting a biochemical signalling cascade in the cell. This is a relatively undirected approach, however, since, in many cases, the drugs’ targets are located throughout the entire brain or even in other parts of the body – not just in the specific area of brain that the drug is meant to act on.
Yanik therefore has a rather different idea of what brain disorder therapies might look like in the future. “Right now it’s nothing more than a vision!” he says with a grin. But it’s a vision he clearly takes seriously: he recently made a successful application to obtain EU funding for the project.
His idea envisages a person lying in bed with their head on a smart pillow containing sensors that communicate wirelessly with microchips implanted in the patient’s cerebral cortex. As the patient sleeps, thousands of tiny embedded electrodes transmit high- resolution information on the activity of individual nerve cells to the chips’ processing units. These, in turn, calculate whether the brain circuits are functioning normally or if they are exhibiting pathological activity patterns that require therapeutic intervention.
In the latter case, an implant connected to the bloodstream releases microparticles containing active agents. The chips activate further modules that generate ultrasonic waves and direct them to a specific point in the brain. The particles cluster together briefly at that point and then release the active agents, which successfully normalise the misfiring brain circuits in a highly focused and extremely targeted way.
Though he is still decades away from implementing this kind of system, some pieces of the puzzle are already the subject of major research work and animal trials. One example is the use of focused ultrasound to release active agents. Yanik and his colleagues recently succeeded in using weak ultrasonic waves to cluster and break open microparticles in target brain areas of rats, releasing batches of active agents that have already been approved for clinical applications. Yanik’s team has also developed novel algorithms to detect pathological brain activity patterns and even to fully correct brain disorders in small animals.
The question remains open as to whether humanity really needs this kind of science-fiction scenario to cure brain disorders. Yanik is convinced it does: “Our existing therapies are not up to the job. Forty percent of the most devastating brain disorders remain untreatable. Swallowing pills to treat mental illness or, at best, bombarding the brain with electromagnetic or ultrasound waves is about as effective as trying to repair a supercomputer with a hammer.”